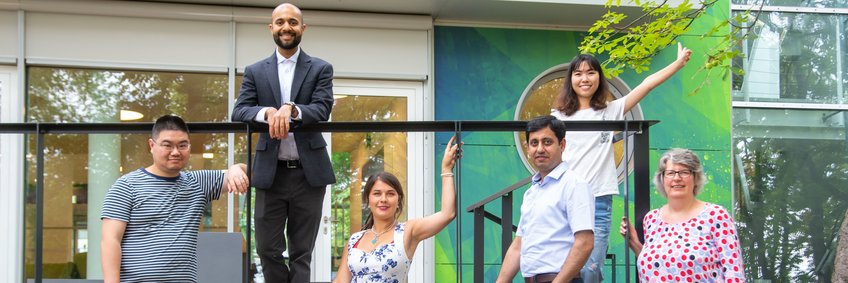
Alexander von Humboldt Sofja Kovalevskaja Group
Group Description
The Alexander von Humboldt Sofia Kovalevskaja Group is led by Dr. Mazhar Ali and began operation in December of 2016. We are working on an interdisciplinary approach to using and exploring properties of topological materials and applying lessons learned from the field of topology in new ways. Our research topics include; investigation of Hall effects and their dependence on Berry curvature and Fermi surface geometry, exploring properties of Josephson Junctions made from topological materials, using topological compounds to assist in the detection of dark matter, and exploring the interplay of frustrated magnetism with unconventional topology in exfoliable 2D materials (including spin liquid candidates).
Spin Hall Effect and Topologically Related Materials
Materials manifesting a large spin Hall effect (SHE) are highly desirable for spintronics applications (ranging from spinFETs to racetrack memory). In particular, materials with high charge to spin conversion efficiencies (SHA) that are able to be fabricated via thin film sputtering are extremely sought after. Only a handful of materials have been shown to have large SHE which are fabricable at scale; Pt/Au-doped-Pt and beta-W/Oxygen-doped-beta-W. Topological Insulators (TI) and Weyl materials also host giant SHE values due to their topological surface states and Berry curvature, respectively. We investigate other topologically related materials; in particular “failed TI’s”, where spin orbit coupling driven gapped Dirac crossings also give rise to large Berry curvature and correspondingly large SHE’s, even though other metallic bands are present. These materials, can be deposited in thin film form via sputtering at room temperature and incorporated into large scale device fabrication. We both carry out electronic structure calculations to look for the particular band structure features which give rise to the large spin Berry curvature as well as fabricate thin films and devices of the relevant materials in our lab to test our theoretical predictions.
Dark Matter Detection using Magnetically Doped TIs
Astrophysical and cosmological observations provide strong evidence for the existence of non-baryonic dark matter (DM). Among possible candidates are dark axions (DA), hypothetical particles suggested to solve the CP problem in quantum chromodynamics (QCD). Searching for the DA is challenging due to its weak coupling to ordinary matter (e.g. photons). It is believed that the earth lies in a local DA field, which has an unknown axion mass of ma and a decay constant fa. It is also believed that ma lies in the few meV regime and the axion coupling lies between 10-12 and 10-14 GeV-1. Currently, no experiment has been created or proposed to probe that mass and coupling; it is a highly sought after range.
It is known that breaking time reversal symmetry in topological insulators can result in so-called “axionic insulators”; insulators which host axionic quasiparticles. A dynamical axionic insulator has 3 parts: 1.) a Chern-Simons action (E dot B) 2.) Electrons governed by the Dirac equation and 3.) a tunable axion mass via an external field. We investigate antiferromagnetic (AFM) TI’s as dynamical axionic insulators which can couple to the DA and explore novel ways to detect axionic dark matter in the previously mentioned mass/coupling range.
2D and Topological Materials; thin flake and JJ properties
“2D” materials have a broad range of potentially new and fascinating physics to explore as well technological applications to be realized. In particular, 2D topological materials like Graphene and WTe2 have once again take the center stage of the condensed matter field as recently superconductivity was shown in bi-layer twisted graphene and the quantum spin Hall effect was seen in WTe2 up to 100K. Also, new, 2D and exfoliable spin liquid candidate materials, like Nb3Cl8, have also become promising candidates to explore in the thin limit for exotic physics.
We explore exotic transport in the geometrically frustrated systems of these spin liquid candidate materials (in the monolayer limit) using our 2D transfer station and fabrication lab. We also fabricate josephson-junctions to explore the nature of induced superconductivity in these materials; particularly in WTe2, where the topological edge states and lack of inversion symmetry results in novel fundamental transport mechanisms.